We research solid-state quantum devices to advance quantum computation, communication and sensing. We study a wide range of systems including superconducting quantum circuits, color centers, integrated photonics and phononics. We develop integrated devices to enable future quantum technologies, and use these systems to gain new insights into quantum dynamics and coherence in nanoscale systems.
We are a highly interdisciplinary group with researchers from electrical engineering, physics, and materials science backgrounds. We are always looking for talented and motivated students and postdocs to join our group.
Current research directions
Please check out our publications and recent recorded talks to learn more about our work.
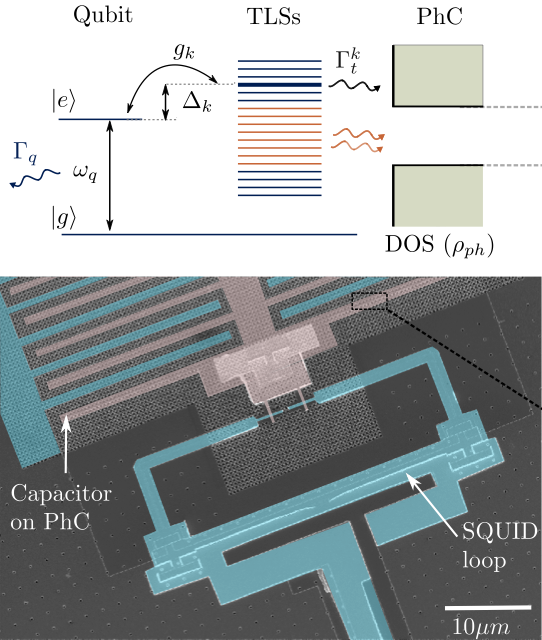
Next-generation superconducting quantum devices
Superconducting quantum circuits are currently the leading solid-state quantum computing platform. Despite remarkable progress, we are yet to fully understand and control dominant dissipation mechanisms that lead to high physical qubit error rates. We study and engineer how superconducting qubits interact with defects and phonons to enable higher-coherence qubits, quantum transducers, and quantum memories. Recent topics include:
- Next-generation superconducting qubits via phononics [Ref. 1]
- Quantum transduction between superconducting qubits, phonons, spins, and optical photons [Ref. 2]
- Microscopic origins of dielectric loss [Ref. 3]
- Coherence in electromechanical quantum systems [Ref. 4]
- Materials origins of qubit-phonon interactions [Ref. 5]
Quantum photonics with spin-photon interfaces in silicon
Silicon is the ideal material platform for building electronic and photonic circuits at scale. We are developing quantum nonlinear silicon photonics that will enable deterministic interactions between single photons and spin qubits for quantum communication and computation. To achieve this, we are developing spin-photon interfaces in integrated silicon photonics:
- Color centers in silicon: How do we design and engineer an atom-like qubit in silicon [Ref1], [Ref2]
- Device integration of color centers to realize high-fidelity spin-photon and photon-photon interactions in silicon photonics [Ref3]
- Demonstration of quantum communication and repeater protocols with silicon photonics at the UC Berkeley – LBL Quantum Network Testbed
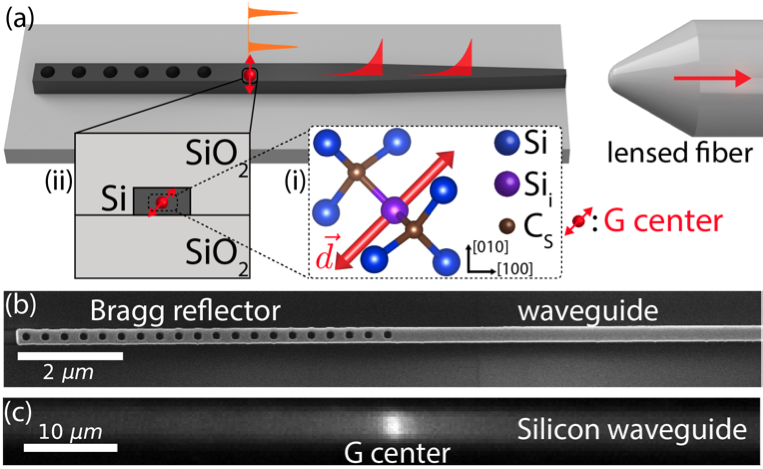